For a list of Prof. Ratcliff’s publications, check the “Publications” section, or his Google Scholar profile.
Evolution of multicellularity
The evolution of multicellular organisms from unicellular ancestors was critical to the evolution of large, complex organisms. While multicellularity has evolved more than 25 times independently during the last 3.5 billion years on Earth, the first steps in this transition remain poorly understood. In our lab, we use experiments and theory to understand:
- Under what conditions does multicellularity evolve?
- How do dumb clumps of cells evolve into bonafide multicellular organisms, complete with developmentally-regulated life cycles, cellular differentiation, and behaviors that break ancestral trade-offs?
- What are the biophysical limitations faced by early multicellular organisms, and how are they solved?
- How do early multicellular life cycles arise, and what are their ecological and evolutionary consequences?
- How do cells evolve into mutually-interdependent parts of a multicellular organism? What prevents multicellular organisms from reverting back to unicellularity?
Our main model system is “snowflake yeast”, which we originally obtained from a unicellular genotype of Baker’s yeast (strain Y55) simply by selecting for rapid sedimentation through liquid media.
Snowflake yeast have an emergent multicellular life cycle, in which daughter cells fail to separate from their mothers, resulting in the growth of branched groups. These groups grow, until strain resulting from cell division severs a cell-cell connection, resulting in the release of a propagule. It turns out that novel multicellular traits, like size at reproduction, emerge from mutations affecting cell-level traits, and are highly heritable. Across generations of growth and selection for larger size, snowflake yeast evolve.
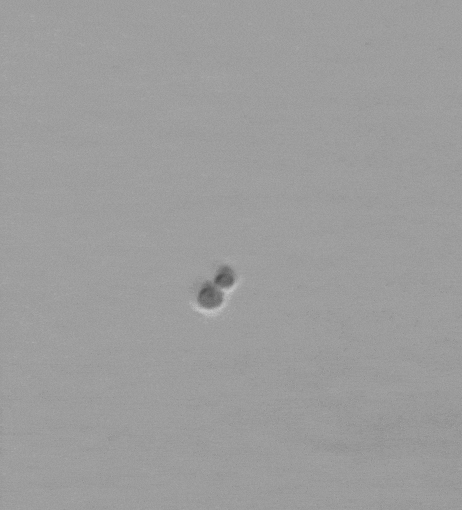
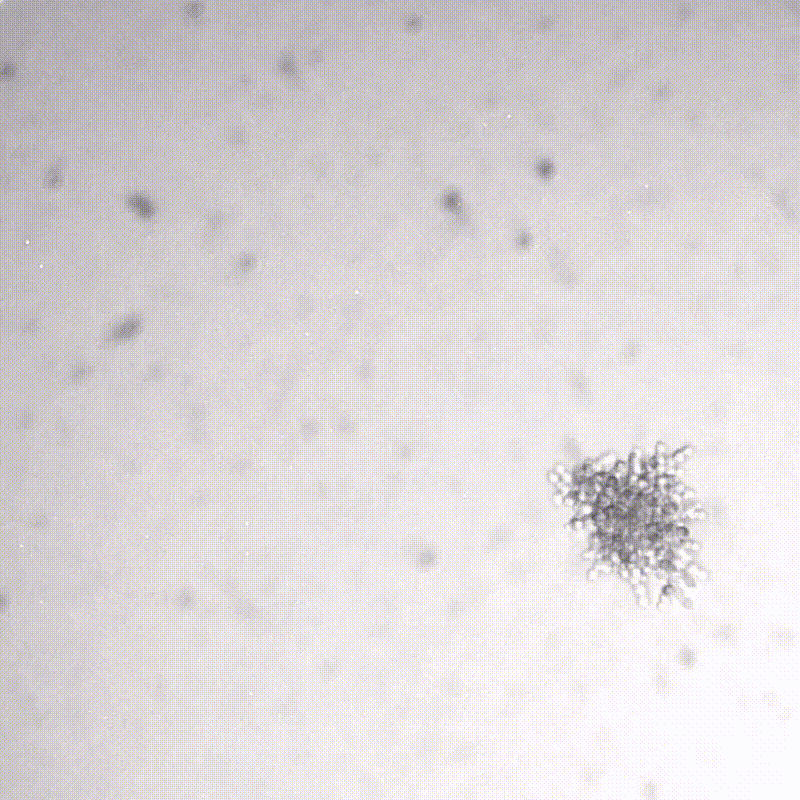
We are currently running a Lenski-inspired Multicellularity Long Term Evolution Experiment (MuLTEE), which we hope will continue for at least the next 25 years. So far, we have currently put snowflake yeast through >1,000 rounds (~5000 generations) of selection for larger group size, evolving multicellular yeast that are ~20,000 times larger than their ancestor. These individual snowflake yeast remain clonal and are visible to the naked eye (larger than fruit flies). They have accomplished this feat through sustained biophysical adaptation, evolving far more elongated cells that ‘entangle’, increasing their mechanical toughness by over 10,000-fold. Individual snowflake yeast evolve from being weaker than gelatin, to as strong and tough as wood. You can read more about this work here. In this system, we are investigating the genomic causes and consequences of multicellular evolution, the origin of multicellular development, emergent fluid flows, and are examining how multicellularity becomes entrenched, preventing reversion (see current projects).
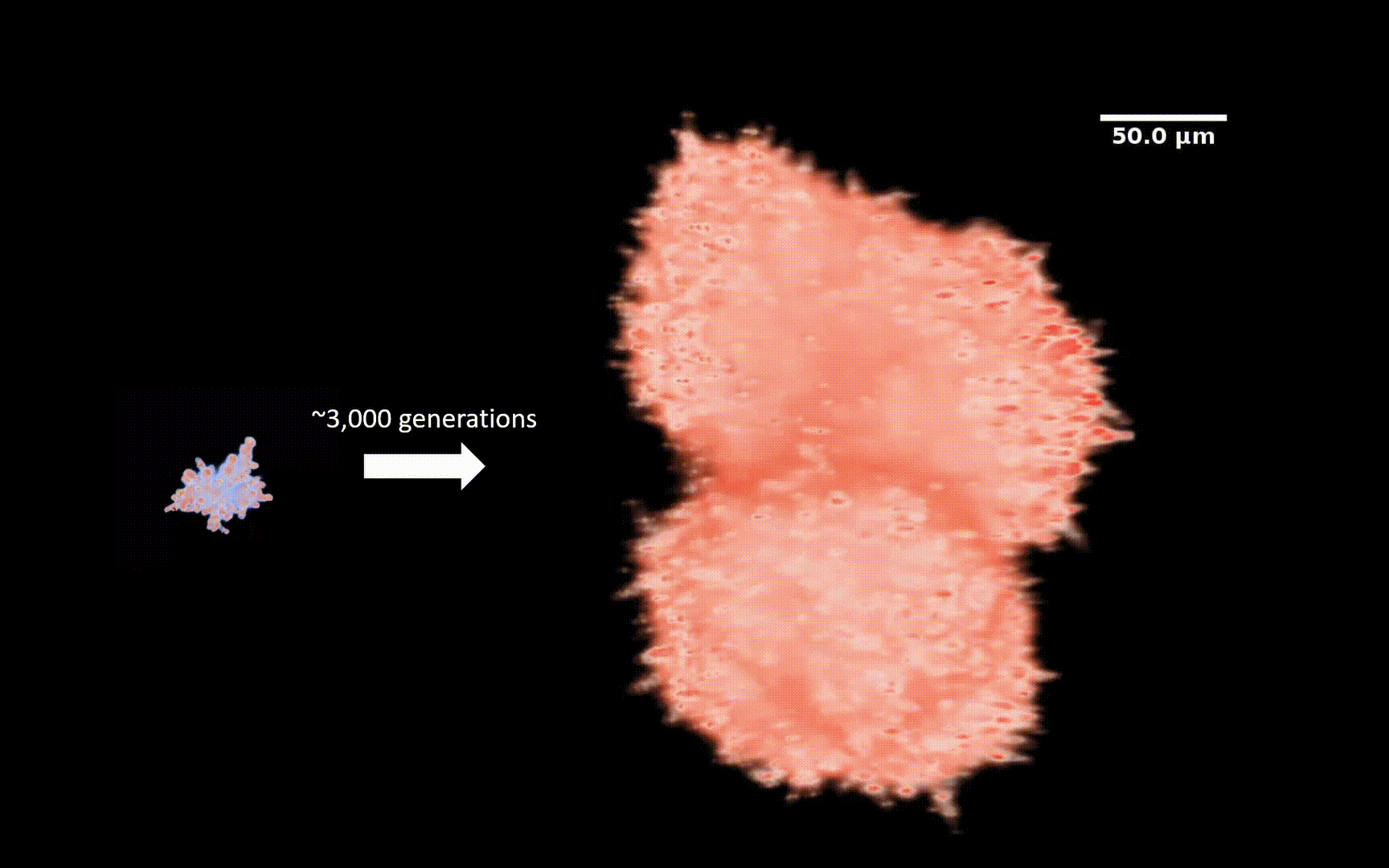
Spatial dynamics of microbial cooperation and conflict
Multicellularity is just an extreme form of social evolution, which we are also quite interested in. Specifically, we study the role of spatial structure in microbial social evolution. The bacterium Vibrio cholerae forms surface-attached biofilms, which it fiercely defends with a ballistic weapon called the Type 6 Secretory System (T6SS) that kills competitors but not clonemates. We showed that this kind of preferential killing strongly drives a spatial phase transition, separating well mixed competitors into clonal domains. Interestingly, by creating strong clonal assortment, this antagonism is a powerful driver of within-strain cooperation. We also showed that cholera can acquire new T6SS alleles through horizontal gene transfer, which is adaptive when the efficacy of current weapons against future competitors is uncertain.
Evolutionary theory
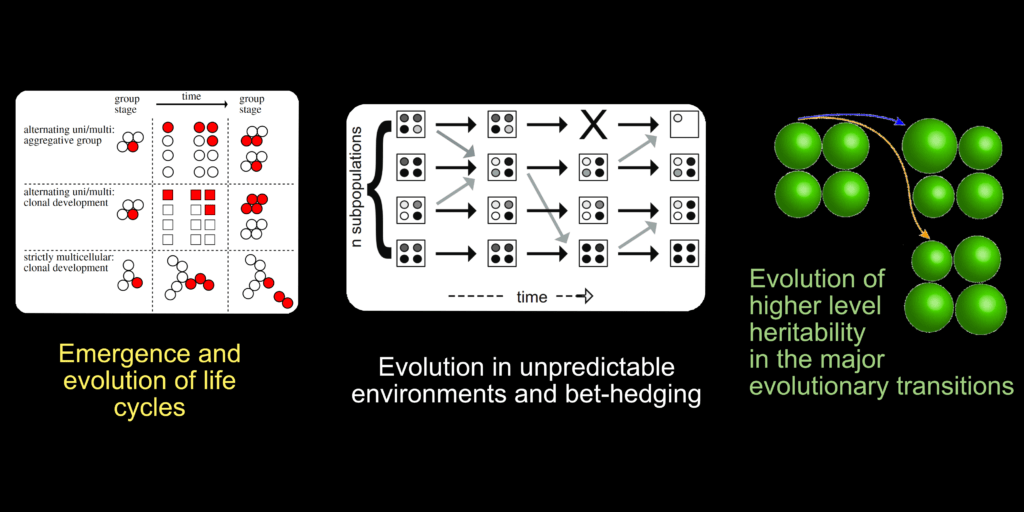
We are interested in how multicellular life cycles emerge and evolve, leading to the evolution of higher-order heritability. Additionally, we are interested in general aspects of evolutionary theory, in particular bet-hedging and the evolution in unpredictable environments.
We are extremely grateful to the National Science Foundation (DEB and IOS), the National Institutes of Health, NASA Exobiology, the Human Frontier Science Program, the Packard Foundation, the Keck Foundation and the Simons Foundation for supporting our research!
